Introduction
Mobile Network Operators (MNOs) are preparing for the rapid deployment of 5G networks. Their aim is to evolve today’s mobile broadband services, through lower unit costs and improved end-user performance, and to address new business segments using 5G system capabilities. To achieve this, operators are designing network architectures that will scale to devise and traffic densities far beyond what is commonplace in LTE networks today and meet the latency and reliability requirements of demanding new service types. This is critical preparation for the 5G super-cycle that will enable operators to extend their reach into diverse markets over the next 10 years.
An essential part of the new design is the transport network that provides connectivity between radio sites, edge data centers, and cloud applications.
Current Transport Architectures for MNOs
Just as mobile services and technologies have evolved from circuit-switched voice services to packet-technology-based data and voice services, the
transport network has evolved rapidly from a purely Time Division Multiplexing (TDM) infrastructure to a packet-based infrastructure.
There are many transition initiatives in the transport domain like CISCO Unified Multiprotocol Label Switching (MPLS) solution, designed around standards-based packet technologies and carried over a high-capacity optical infrastructure. Globally, mobile operators have comprehensively adopted Unified MPLS, setting the standard for IP-based mobile service delivery.
But the question now, Why do we need a new approach?
Although extremely successful and widely deployed, there are complexities to the Unified MPLS architecture when deployed in extremely large networks. These revolve around the complexity of the control plane, the number of control-plane protocols, and the amount of device-level configurations required when building a service that spans many domains.
The introduction of 5G introduces additional challenges, which include:
- Footprint expansion due to the densification of the radio.
- Need to incorporate the network or edge data center seamlessly into the transport network.
- Use of Virtualized Network Functions (VNF) for radio and mobile core functions.
- Network slicing.
- The control plane and user plane separation introduced by the CUPS architecture.
5G network requirements
The 3GPP standards organization and 5G industry developers are specifying the main features of 5G. The below figure outlines the functionality already specified and to be available in upcoming 3GPP releases.
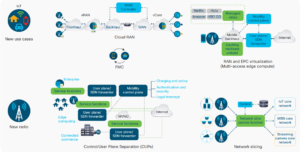
Source: CISCO
5G Transport Domain
The transport domain delivers connectivity between remote sites and equipment/devices. Backhaul serves both ends of the transmission – for example, to connect a base station (BS) to an access network or a centra office – while fronthaul is a term used when the BS antennas are connected to a remote integrated radio frequency (RF) unit, or to a centrally located baseband (BB) unit. In addition to providing bulk connectivity for the operator’s mobile network fronthaul and backhaul.
5G RAN technology puts new requirements on the bandwidth and latency of transport networks. Consequently, a high degree of automation and coordination within and cross-network domains will be required. A concept known as RAN transport interaction (RTI) introduces coordination between the radio, transport, and packet core layers of an operator’s mobile network, providing network-wide optimization and service assurance. Examples of such coordination include:
- Support for various industries extending network resource differentiation into the transport network.
- Proactive congestion management, enabling transport aware RAN load balancing for improved user QoE.
- Securing fairness between radio technologies within the transport network.
5G RAN architectures
In order to support the changing nature of the 5G network, it is clear that one of the most important factors to address is the current and future architecture of the RAN, the link that the radio sites use to connect to the transport network. Not only is this where operators spend most of their money today, but being geographically dispersed, it is also difficult to manage. Therefore, any development or evolution of the RAN to improve the efficiency or reduce the operational costs requires serious consideration.
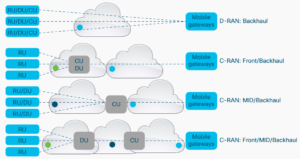
5G RAN Architectures
Traditionally, we implemented the RAN as a Distributed RAN (D-RAN) architecture, where all the radio functionality (the NodeB or eNodeB) resided in the cell site, which then exchanged IP packets with the mobile core via the backhaul network.
The IP protocol was used to carry the traffic streams, and the data rates needed were roughly equivalent to the combined user data rates of user equipment that was utilizing the radios hosted in that cell site. With 4G, and more so with 5G, an alternative to the D-RAN is the disaggregated or Centralized RAN (C-RAN), whereby the upper layers of the radio functions are separated from the lower layers and moved to a shared, centralized location.
Mapping 5G RAN to Transport Network
The transport network is especially important because it provides connectivity between the NG-RAN functional modules and therefore determines, in the first instance, which deployment topologies are possible, and then, which are cost-effective. The below figure shows the primary functional elements of the 5G RAN (RU, DU, and CU) and how they are logically mapped to the transport network.
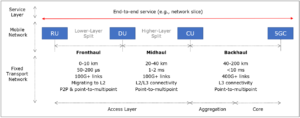
Source: Heavy Reading
References:
- Ericsson,5G systems – Enabling the transformation of industry and society.
- Huawei, 5G Network Architecture.
- Nokia, Designing the transport network for 5G.
- Cisco, Converged 5G xHaul Transport.
- Heavy Reading, New Transport Network Architectures for 5G RAN.